Articles List 21-26 Dec 2017
- Emma
- Dec 26, 2017
- 8 min read
In addition to reading more news sites and blogs on robotics, I have decided to read more journal articles as well. I have heard that reading research papers is itself a skill which I would like to develop. Right now I plan to focus mainly on robotics, but you can already tell from the list for this week that I have not exclusively read about robotics, which is good - more learning is better!
Cui LJ, Li DC, Liu J, Zhang L, Xing Y. Intraocular pressure control of a novel glaucoma drainage device - in vitro and in vivo studies. Int J Ophthalmol 2017; 10(9): 1354-1360.
This article describes a new embodiment of an artificial trabeculum drainage system (ATDS) that draws on the Ahmed valve and other drainage devices currently used in trabeculectomies. The team modeled the expected pressure drops across the device using Bernoulli's principle and Poiseuille flow and then compared their model to results obtained from testing the new device in a manometer closed system. Finally, 20 devices were implanted in 20 rabbits (one eye had the ATDS, the other was a control) and the eyes were monitored for 60 days before enucleating (i.e. surgically removing the eyes posthumously). The study showed that the devices caused a significant drop in IOP but more testing was needed to understand and mitigate the complications due to the devices' implantations. (I was a little rusty on Bernoulli's principle and Poiseuille flow so I wrote a quick blog post to explain them.)
Guadana R, Ananthula HK, Parenky A, Mitra AK. Ocular drug delivery. The AAPS Journal 2010; 12(93): 348 - 360.
This article was a survey of different drug delivery methods to different areas of the eye. It is a little outdated (published in 2010) so there may be new developments since this paper was written. I focused on some particular information that I found most interesting, such as the fact that intravitreal drug distribution is non-uniform; small molecules diffuse quickly, larger ones diffuse slowly. The authors did not touch on intracameral drug distribution in as much detail (i.e. drug delivery to the anterior rather than posterior chamber) but they did mention that intracameral drug distribution can be advantageous over topical application of drug because it bypasses the corneal barrier.
The authors listed some interesting facts on topical drug delivery. Human tear film volume is on average 7 uL. The cul de sac (i.e. where the tear duct is located) can hold about 30 uL of fluid from an eye drop. The tear film is completely replaced every 2-3 minutes, but most drug is washed away in even less time, approximately 15-30 seconds. As a result, less than 5% of an eye drop dose actual penetrates through the cornea and reaches intraocular tissue.
Neovascularization is when new blood vessels start to grow in the innermost layer of the choroid, often in response to ischemia (inadequate blood supply). Neovascularization can cause glaucoma because the vessels can block the aqueous humor outflow pathway (through trabecular meshwork to Schlemm's canal) - this is called neovascularization glaucoma.
Source: https://en.wikipedia.org/wiki/Neovascularization
Melanin in the iris and other pigmented tissue (uvea) can bond with drug and affect drug delivery and drug response, requiring larger doses.
Other ways to convey drug effectively are transporters (membrane-bound proteins that help deliver drug to a target site), colloids used to dose the eye with drug (sustained drug release), drug coated micro needles (500 - 750 um in length), enhancing corneal penetration of drugs using ultrasound (20kHz for 1 hour), iontophoresis (using electrical current to introduce drug). It can be very difficult to impossible to deliver drug topically and enable it to reach target tissue in the posterior chamber of the eye.
Lee JH, Pidaparti RM, Atkinson GM, Moorthy RS. Design of an implantable device for ocular drug delivery. Journal of Drug Delivery 2012; Article ID 527516.
This article described the construction and early testing of an implantable drug delivery device for treating eye diseases. The device uses passive diffusion to delivery drug to the eye. The authors designed this device for the posterior chamber of the eye in order to treat diseases such as age-related macular degeneration (AMD). AMD is a leading cause of blindness in the US and is characterized by blurred or loss of vision in the center of the visual field. The wet form of AMD is where neovascularization occurs in the choroid. Since the blood vessels formed under neovascularization are new and fragile, they can leak or break, causing blood to pool under the macula (at the center of visual field, near focal point on the retina). Wet AMD is only found in 10-20% of cases of AMD. Dry AMD is more common, and is usually the early phase of the disease and accounts for 80-90% of cases. Dry AMD can become an advanced form of the disease, and in that case it is called geographic atrophy.
Source: https://en.wikipedia.org/wiki/Macular_degeneration
This paper (and many others that I read this week) define 3 primary categories of ocular implants:
biodegradable or non-biodegradable
atypical implantable pump systems
implantable pump systems
Implantable pumps can control rate and volume of drug delivery, using peristaltic, osmotic or infusion technology.
Peristaltic pumps squeeze fluid through a tube as shown in the illustration below.
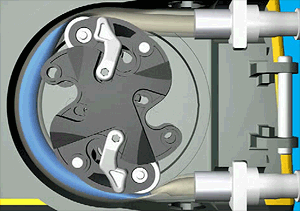
Source: Illustration by Njmcca - Own work. Previously published: http://www.watson-marlow.com/, CC BY-SA 3.0, https://commons.wikimedia.org/w/index.php?curid=26794077
Retisert is an implantable drug capsule that is anchored to the sclera via a suture in the posterior chamber.
Source: http://www.retisert.com/surgical-procedure
Lee et al uses Fick's Second Law to describe how drug diffuses through the posterior chamber from their drug delivery device. Their device uses micro/nano channels for drug delivery, and is also attached to the sclera with sutures. The device is intended to be refillable so that the patient does not have to go back to the surgeon for frequent device replacement surgeries. The drug is stored in a PDMS reservoir and flows through "nanomicrochannels" coated in hydrophilic material to the exit point of the device. The authors developed a device with a total capacity of 280 uL of drug. Their target elution rate is 0.07 nL/min. This drug load theoretically extends out to 2800 days (7 years) if we assume constant elution of 0.07 nL/min (equivalent to 0.1 uL/day). However, the authors estimated a lower product lifespan after modeling the drug diffusion through the device.
Lee et al used ANSYS Multiphysics to model the drug diffusion through the channels of the device with an equivalent thermodynamics model. They calculated the steady state diffusion rates for each of 6 microchannel designs with the simulation software. In general, full or steady flow rates were achieved around 150 seconds. The flow rates varied around 0.07 nL/min which is why the expected lifespan of the device was not as long as 7 years.
Lo R, Li PY, Saati S, Agrawal RN, Humayun MS, Meng E. A passive MEMS drug delivery pump for treatment of ocular diseases. Biomed Microdevices, 2009; DOI 10.1007/s10544-009-9313-9.
Lo et al describe another kind of passive MEMS device for delivering drugs to the eye. This device uses pressure applied by the patient's finger to force drug from a reservoir into either the posterior or anterior chamber of the eye. They explain that the half life of drugs in the vitreous cavity can be short enough to require intraocular injections 1-3 times/week. Most delivery devices must be able to operate under normal pressure conditions (approx 15 mmHg) and higher for glaucoma patients(approx 22 mmHg), as well as other high pressure scenarios, like flying or sneezing.
According to Lo et al, 5 types of pumps have been investigated for ocular drug delivery, namely:
peristaltic
positive displacement
infusion
osmotic
controlled release micropumps
Also according to Lo et al, space considerations inside of the eye have made these 5 types unsuitable for ocular implants. Instead, the team proposes using a microreservoir (leveraging MEMS technology) because they have good repeatibility and controlability. Microreservoirs can also seal different drugs in different capsules that break down due to electrochemical dissolution to release combinations of different drugs. Lo et al were targeting a 10uL/min flow rate in their design of a refillable, biocompatible implant with a control to prevent overdosage using MEMS technology. (Note that other papers, such as Saati et al below, targeted lower flow rates of 2 uL/min in the anterior chamber.) The device would have a minimum lifetime of 2 years, and should be refilled no more than once per month. The mode of delivery requires the patient to apply force with a finger to the drug chamber to force it out through a check valve into the eye. The "cracking pressure" of the valve is set to prevent backflow of ocular fluid into the device, and to prevent drug from spilling into the eye unintentionally in high pressure scenarios. The device will be sutured to the outside of the eye between Tenon's Capsule and the eyeball. The device can be refilled using a non coring needing (which tears the PDMS and allows it to self seal; a coring needle would punch a hole in the device that would not seal). The device has additional mechanical features to prevent the needle from puncturing the entire device and to help the surgeon visualize and refill the device. Two embodiments were developed with volumes of ~40 uL and ~60 uL.
Lo et al performed testing to:
characterize the closing time of the check valve
determine the "cracking" pressure of the check valve
determine the maximum number of times the same point on the PDMS device could be pierced with a needle to refill
evaluate the bond strength between the PDMS layers after different treatments such as oxygen plasma or wet chemical treatment
The team found that it was necessary to shorten the closing time of the check valve as much as possible to prevent accidental dosing; this embodiment did not resist transient pressures from sneezing or flying, for instance. These tests were performed in bench studies, an ex vivo study with pig eyes and an in vivo study with rabbits.
Saati S, Lo R, Li PY, Meng E, Varma R, Humayun MS. Mini drug pump for ophthalmic use. Trans Am Opthalmol Soc 2009; 107: 60-71.
Saati et al used the same ocular drug delivery platform in this article as was described in the previous article by Lo et al. This paper focused on developing two different activation methods - manual activation (squeezed) and electrical activation (electrolysis occurs which electrolyses water and the released hydrogen/oxygen forces the valve open so that drug is released in a bolus).
I was not sure what would happen to the gases formed during electrolysis so I looked up how electrolysis works. It is a reversible chemical reaction; once the oxygen and hydrogen gases are formed, they can react to form water (and generate some energy in the process). I am still not sure where this excess energy would go or if it could damage the eye. I also wonder if there is a risk of releasing the gases into the eye and forming a bubble.
Source: https://en.wikipedia.org/wiki/Regenerative_fuel_cell
Source: https://en.wikipedia.org/wiki/Electrolysis_of_water
The electrolysis process is current controlled because this is directly correlated to the volume of gas produced and the drug flow rate out of the device. The device can deliver a bolus of drug by applying a square wave of electricity, or it can perform continuous release by continuously applying current to the device (either directly via batteries or via wireless inductive power transfer).
Saati et al performed experiments to determine their device's:
electrolysis performance
pump efficiency
bolus vs continuous delivery
gas recombination
back pressure
Saati et al also performed ex vivo testing in enucleated pig eyes and in vivo testing in rabbit eyes. They were able to release fluid at a rate of 2.0 uL/min using 0.4mW, using currents ranging from 5 uA to 1.25 mA. According to Saati et al, the natural secretion rate of aqueous humor from ciliary body into anterior chamber is approximately 2.4 +/- 0.6 uL/min, so they targeted a drug delivery rate of less than 2.0 uL/min. Depending on current delivered, a dose of 250 nL could be sent in 2.36 s for 1.0mA and 1.75 s for 1.5mA.
The second generation prototype will separate the chamber for electrolysis from the drug load via a flexible diaphragm, because Saati et al wanted to prevent oxidation of drug which affects pH, and prevent gases from escaping into the eye.
Comments